Looking for an eco-friendly cooling solution? Discover how the little-known heat exchanger for thermoacoustic refrigeration is changing the game for homeowners!
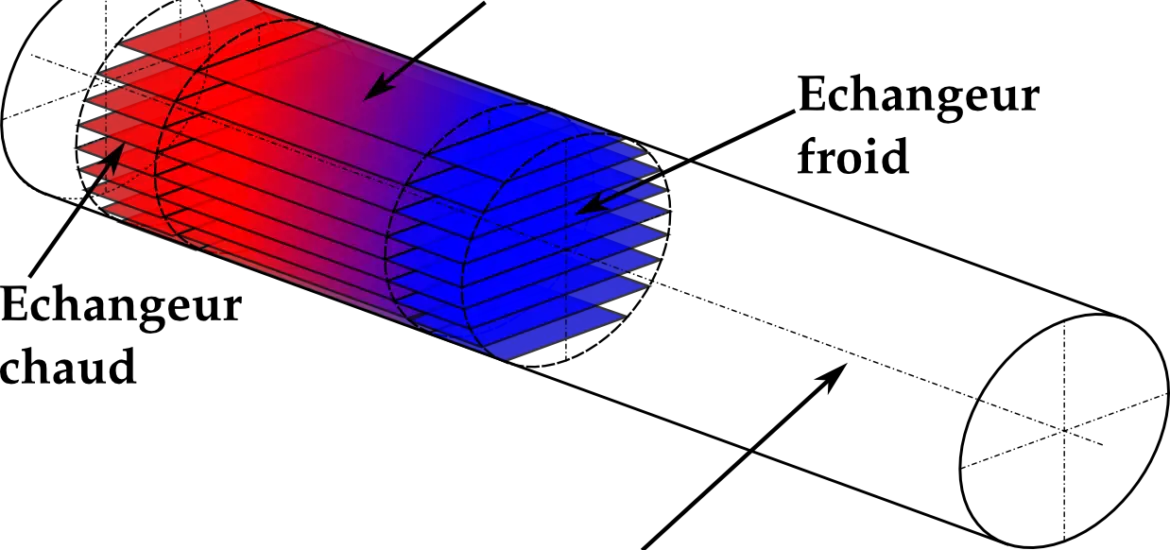
Table of Contents
Thermoacoustic refrigeration overview
Thermoacoustic refrigeration uses sound waves to move heat, eliminating the need for harmful refrigerants. A perfect choice for eco-conscious homeowners!
Principles of thermoacoustic refrigeration
Sound waves create temperature gradients, causing heat to flow from hot to cold zones. Oscillating gas particles carry heat along, making this magic happen.
Advantages and disadvantages of thermoacoustic refrigeration
It’s eco-friendly, with zero ozone depletion and reduced global warming impact. But it can be pricey and bulkier compared to conventional systems. There’s always a trade-off!
Role of heat exchangers in thermoacoustic refrigeration
Heat transfer in thermoacoustic systems
Heat exchangers are vital for transferring heat efficiently, ensuring smooth operation of thermoacoustic refrigeration.
Types of heat exchangers used in thermoacoustic refrigeration
Various heat exchangers, like shell and tube, plate, finned tube, and compact ones, are used. Each has its pros and cons, but all are essential for success!
Heat Exchanger Types
Shell and tube heat exchangers
Design and construction
A classic design, with tubes inside a larger shell. Fluids flow inside the tubes and shell, transferring heat through the tube walls. Simple, yet effective!
Applications in thermoacoustic refrigeration
Shell and tube heat exchangers work well for large thermoacoustic systems, providing efficient heat transfer and easy maintenance.
Advantages and disadvantages
They’re robust and reliable, but can be bulky and heavy, making them less suitable for smaller applications.
Plate heat exchangers
Design and construction
These exchangers use thin, corrugated plates stacked together, with fluids flowing between them. Compact and efficient!
Applications in thermoacoustic refrigeration
Plate heat exchangers are perfect for smaller thermoacoustic systems, where space and weight matter.
Advantages and disadvantages
They’re compact and efficient, but can be challenging to maintain and clean. You win some, you lose some!
Finned tube heat exchangers
Design and construction
These exchangers have tubes with fins attached, increasing heat transfer area. More fins, more heat transfer!
Applications in thermoacoustic refrigeration
Finned tube heat exchangers are excellent for medium-sized thermoacoustic systems, offering enhanced heat transfer in compact spaces.
Advantages and disadvantages
They boast high efficiency and compactness, but their complex design can make cleaning and maintenance a bit tricky.
Compact heat exchangers
Design and construction
Compact heat exchangers are designed for minimal space and weight while maintaining high heat transfer efficiency. Perfect for tight spots!
Applications in thermoacoustic refrigeration
These exchangers are ideal for small, space-constrained thermoacoustic systems, where every inch counts.
Advantages and disadvantages
Compact heat exchangers are space-saving and lightweight, but their small size may limit their heat transfer capacity.
Heat Exchanger Design and Optimization
Design considerations
Thermoacoustic engine requirements
Heat exchanger design should meet the specific requirements of the thermoacoustic engine, ensuring efficient heat transfer and seamless integration.
Material selection
Choosing the right materials for heat exchangers is crucial, as they impact thermal performance, durability, and cost. Don’t skimp on quality!
Thermal performance
Optimizing heat exchanger design for maximum thermal performance is key, ensuring that the thermoacoustic system operates effectively.
Pressure drop and flow distribution
Minimizing pressure drop and ensuring even flow distribution are essential for efficient heat transfer and system performance.
Optimization techniques
Computational fluid dynamics (CFD)
CFD simulations help predict and optimize heat exchanger performance, saving time and resources during the design process.
Genetic algorithms
These algorithms use evolutionary principles to optimize heat exchanger designs, finding the best solution for specific applications.
Multi-objective optimization
Considering multiple objectives, like cost, size, and performance, ensures that heat exchanger design is well-balanced and effective.
Fabrication and Assembly
Manufacturing processes
Machining
Machining can be used to produce heat exchanger components with high precision and accuracy, ensuring a perfect fit.
Casting
Casting is a cost-effective method for producing heat exchanger components, especially for large-scale production.
Additive manufacturing
3D printing allows for rapid prototyping and complex geometries, enabling innovative heat exchanger designs.
Assembly methods
Brazing
Brazing can be used to join heat exchanger components, creating strong, leak-free connections.
Welding
Welding offers a durable and reliable method for assembling heat exchangers, ensuring long-lasting performance.
Mechanical fastening
Mechanical fasteners, like bolts and screws, can be used to assemble heat exchangers, allowing for easy disassembly and maintenance.
Performance Evaluation and Validation
Experimental setups
Test rigs
Test rigs are essential for evaluating heat exchanger performance, simulating real-world conditions and measuring critical parameters.
Instrumentation
High-quality sensors and instruments are used to accurately measure temperature, pressure, and flow rates, ensuring reliable performance data.
Performance metrics
Coefficient of performance (COP)
COP measures the efficiency of a heat exchanger, comparing the amount of heat transferred to the energy input. Higher COP, better efficiency!
Heat transfer effectiveness
This metric evaluates how effectively a heat exchanger transfers heat, considering factors like temperature difference and heat capacity rates.
Pressure drop
Measuring pressure drop helps determine the impact of a heat exchanger on the overall system, ensuring smooth and efficient operation.
Validation and uncertainty analysis
Comparison with numerical simulations
Comparing experimental data with numerical simulations helps validate heat exchanger performance and identify areas for improvement.
Uncertainty quantification
Assessing uncertainties in experimental data ensures reliable performance evaluation and supports confident decision-making.
Applications and Future Prospects
Thermoacoustic cooling systems
Domestic appliances
Thermoacoustic refrigeration can be used in eco-friendly home appliances, like refrigerators and air conditioners, reducing environmental impact.
Industrial processes
From food processing to electronics manufacturing, thermoacoustic cooling offers energy-efficient solutions for various industries.
Waste heat recovery
Power generation
Thermoacoustic systems can convert waste heat into usable power, enhancing overall energy efficiency and sustainability.
Desalination
Using waste heat for thermoacoustic-driven desalination can provide clean water with minimal environmental impact. A win-win!
Environmental impact and sustainability
Ozone depletion potential (ODP)
Thermoacoustic refrigeration has zero ODP, making it a responsible choice for homeowners concerned about the environment.
Global warming potential (GWP)
With no harmful refrigerants, thermoacoustic systems have a significantly lower GWP compared to conventional cooling technologies.
Energy efficiency
By optimizing heat exchanger performance, thermoacoustic systems can provide energy-efficient cooling solutions for a greener future.
Conclusion
Summary of key findings
Heat exchangers play a pivotal role in thermoacoustic refrigeration, offering an eco-friendly cooling alternative for homeowners.
Future research directions
As research and advancements continue, thermoacoustic refrigeration may become the go-to solution for eco-friendly cooling. The future looks cool!